INSTRUMENTATION
[Photoacoustic imaging systems] [Photoacoustic-photothermal probe] [Laser diode excitation system] Introduction
Our activities encompass the development of novel photoacoustic detection and excitation instrumentation. The former includes the development of a range of 3D multiwavelength photoacoustic scanners for non invasive, short range, high resolution tissue imaging. Additionally, miniature photoacoustic-photothermal probes for minimally invasive use such as insertion via a biopsy needle or endoscope As well as detection instrumentation, portable low cost multiwavelength laser diode excitation systems for clinical use are being developed as an alternative to current OPO based laser systems.
Backward-mode planar photoacoustic scanner
An all-optical multiwavelength photoacoustic scanner has been developed for short range (<1cm) high resolution (10-100µm) 3D imaging. Applications include visualising structural and functional abnormalities in the microvasculature such as those characteristic of tumours or soft tissue damage such as burns or ulcerations. The system comprises a tunable (410-2100nm) fibre coupled OPO laser system that provides 10ns optical pulses for generating the photoacoustic signals and a transparent planar Fabry Perot (FP) polymer film ultrasound sensor for detecting them. The sensor is placed in acoustic contact with the surface of the target tissue, the excitation laser pulses transmitted through it and the resulting photoacoustic signals recorded at different points over the surface of the sensor. From the time-of-arrival of the signals, and with knowledge of the speed of sound, a 3D image of the tissue structure, based upon the absorbed optical energy distribution, can then be reconstructed. This type of imaging instrument has several important advantages over conventional piezoelectric based photoacoustic detection systems. Firstly, the system operates in “backward mode”. That is to say, the photoacoustic signals can be detected on the same side and over the same region of the tissue surface that is irradiated with the excitation light, a consequence of the transparent nature of the sensor. This is particularly important for imaging superficial anatomical features, such as blood vessels in the skin, where it would be problematic to deliver the excitation laser beam around an array of opaque piezoelectric receivers. Secondly, the concept provides excellent acoustic performance, with uniform broadband frequency response characteristics (to at least 30MHz) and wideband detection sensitivities (<0.1kPa noise-equivalent-pressure) comparable to piezoelectric PVDF receivers but with much smaller “element” sizes (<50µm) and “interelement” spacings – the latter being a consequence of the optically addressable nature of the sensor which, in principle, affords near-optical diffraction limited spatial sampling of the incident acoustic field. These attributes make the instrument well suited to high resolution (10µm-100µm) tissue imaging applications - click here to see examples of some of the images that have been obtained with the system. The following provides more details on the FP sensor head design, the optical scanning system used to interrogate it and the performance of the instrument.
Principles of operation: The FP sensor head, shown in figure 1, comprises a wedged transparent polymer backing stub on to which a multilayer sensing structure is vacuum deposited. This consists of a Parylene polymer film spacer (typically 10-50µm, depending upon the acoustic bandwidth required) sandwiched between 2 dichroic dielectric mirrors. These are designed to be highly reflective (>95%) between 1500-1700nm thus forming a high finesse Fabry Perot cavity in this wavelength range but highly transmissive between 600nm-1200nm. The latter enables the excitation laser pulses from the OPO in the near infrared (NIR) window, where biological tissues are relatively transparent, to be transmitted through the sensor head into the tissue (figure 2). The photoacoustic signals generated by the absorption of the laser energy propagate back to the surface where they modulate the optical thickness of the polymer film and thus the reflectivity of the sensing structure in the 1500-1700nm wavelength.
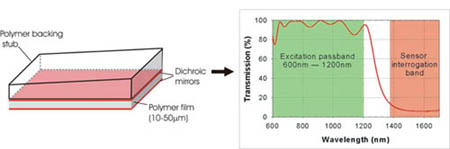 |
|
(a) |
(b) |
(c) |
Figure 1 (a) Backward mode Fabry Perot (FP) polymer film ultrasound sensor. The backing stub is typically 10mm thick and of lateral dimensions 50mm x 30mm. (b) Transmission characteristics of the dichroic dielectric coatings used to form the mirrors of the FP sensing interferometer. The coatings are highly transmissive between 600nm-1200nm enabling NIR excitation laser pulses in this wavelength range to be transmitted through the sensor but highly reflective (95%) between 1500nm-1600nm where they form a high finesse Fabry Perot cavity (c) Photograph of sensor (wedge side uppermost) under narrow band UV illumination illustrating the concentric elliptical FPI transmission fringes and the transparent nature of the sensor head.
By raster scanning a 1550nm focussed laser beam (that is wavelength tuned to optimally set the phase bias at each scan point) over the surface of the sensor as shown in figure 2 and detecting the reflected intensity modulation with a photodiode, the incident time dependent photoacoustic waves arriving at the sensor can be mapped in 2D. In this way the system can be regarded as a notional 2D ultrasound array, the aperture of which is defined by the dimensions of the scan region and the element size and inter-element spacing by the laser spot size and the scan step size respectively. In order to reconstruct a 3D image from the detected time-resolved photoacoustic signals recorded over the surface of the sensor an efficient k-space backpropagation algorithm is used.
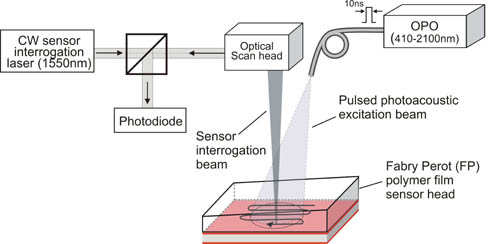
Figure 2 Backward mode multiwavelength photoacoustic scanning instrument. A tunable OPO laser system provides nanosecond optical pulses for generating the photoacoustic signals within the target which is placed underneath and in acoustic contact with the FP sensor head. A second laser provides a focussed interrogation laser beam that is rapidly scanned using a 2D galvanometer based scan head over surface of the sensor to map the photoacoustic signals generated within the target.
System performance: The system can map photoacoustic signals over an area of 50mm x 30 mm in steps of 10µm with a laser spot diameter of 64µm. The minimum point-to-point acquisition time of the scanning system is 10µm, although in practice this is currently limited to 100ms by the 10Hz pulse repetition frequency of the OPO system. The sensor provides a peak noise-equivalent-pressure of 0.1kPa (over a 25MHz measurement bandwidth) and a -3dB bandwidth of 28MHz for a 38µm thick polymer film spacer. The spatial resolution that the system can provide is dependent upon many factors such as imaging depth, total measurement aperture, spatial sampling parameters and so on. As an indication however, PSF measurements show that, for depths of a few mm, vertical and lateral spatial resolutions of 20µm and 50µm respectively are achievable. The system has now been evaluated extensively using tissue phantoms and ex vivo tissues demonstrating that it can provide excellent high resolution (<50µm) 3D images of tissue vascular anatomy. Click here to view these images.
Applications: The high resolution capability of the system makes it particularly well suited to clinical dermatological applications that require visualising the structure and function (via the oxygenation status) of blood vessel networks in the skin. Among these are the assessment of burn depths, wound healing, pressure sores, plastic surgery procedures (grafts, flaps etc.) and characterising skin pathologies: for example, to provide a more accurate diagnosis and staging of tumours such as malignant melanomas and help plan their surgical excision. As well as clinical applications, the system is being evaluated as a investigative tool for undertaking basic research in the life sciences, for example studying the structure and oxygenation status of the microvasculature associated with superficial tumours in order to develop new cancer treatments. More generally, the instrument is intended for use in a variety of molecular imaging applications in cardiovascular biology, preclinical neurology and oncology.
References:
-
Zhang, E, Laufer, J, Beard, P (2008): Backward-mode multiwavelength photoacoustic scanner using a planar Fabry-Perot polymer film ultrasound sensor for high-resolution three-dimensional imaging of biological tissues, Applied Optics 47, 561-577. Download PDF file
-
Zhang E. and Beard PC, Broadband ultrasound field mapping system using a wavelength tuned, optically scanned focussed beam to interrogate a Fabry Perot polymer film sensor, IEEE Transactions on Ultrasonics, Ferroelectrics and Frequency control, Vol 53, No 7, pp13301338, 2006 Download PDF file
-
Zhang EZ and Beard PC, 2D backward-mode photoacoustic imaging system for NIR (650-1200nm) spectroscopic biomedical applications, Proc. SPIE Vol. 6086, pp60860H-1 -7, 2006 Download PDF file
-
Beard PC , Photoacoustic imaging of blood vessel equivalent phantoms, Proc, SPIE, Vol 4618, p54-62, 2002 Download PDF file
-
Beard PC, Zhang EZ, Cox BT, Transparent Fabry Perot polymer film ultrasound array for backward-mode photoacoustic imaging, Proc. SPIE 5320, pp230-237, 2004 Download PDF file
Photoacoustic-photothermal probe
Pulsed photoacoustic and photothermal techniques are investigative methods in which short sub-ablation threshold excitation laser pulses are absorbed in a target absorber producing both acoustic (thermoelastic) and thermal waves. These waves act as carriers of information relating to the optical, acoustic and thermal properties of the target absorber and can be used to describe its constituents and structure. Applications include the characterisation of biological tissue and non-destructive testing of materials and structures. Whilst photoacoustic and photothermal techniques provide an inherently powerful means of characterising a target, their practical implementation can be problematic using conventional acoustic and thermal detection methods. This is particularly so when it is required that the generation and detection of the photoacoustic or photothermal signals take place on the same side of the target as is generally required for the in situ characterisation of biological tissues. In such cases the acoustic/thermal detector should be transparent so that it can be aligned coaxially with the excitation laser beam thus excluding most conventional piezoelectric and pyroelectric contact transducer configurations. Biomedical implementations also often require a miniature, flexible probe type format for minimally invasive use such as insertion via a biopsy needle or endoscope and this too represents a substantial challenge using existing methods. A miniature all-optical probe that employs a transparent acoustically and thermally sensitive Fabry Perot sensor for making photoacoustic and photothermal measurements simultaneously has been developed that offers a potential solution to these limitations.
Figure 1 Schematic of the sensor head probe.
Principles of operation: A schematic of the sensor head probe is shown in figure 1. A 380µm core diameter multimode optical fibre with a transparent Fabry Perot polymer film sensor mounted at its distal end is placed in contact with the target absorber (figure 1). Nanosecond, sub-millijoule optical pulses from a Q switched Nd:YAG laser are launched into the fibre, transmitted through the sensor and absorbed in the target producing thermal waves with a typical duration of the order of a few hundred milliseconds. In addition, rapid thermal expansion occurs generating ultrasonic thermoelastic waves with a typical duration of several hundred nanoseconds. Both thermal and thermoelastic waves are detected by the sensor at the tip of the fibre. The sensor itself comprises a transparent 50μm thick polymer film. This can either be deposited under vacuum directly on to the cleaved end of the fibre using the Parylene process or a discrete polymer film such as PET mounted at the fibre can be used. When illuminated by light launched into the fibre from a CW low power tuneable laser source, the polymer film acts as a low finesse Fabry Perot interferometer with the refractive index mismatches on either side of the film providing the mirrors of the interferometer. An incident thermal or thermoelastic wave changes the optical thickness of the film and hence the optical phase difference between the Fresnel reflections from either side of the film. This produces a corresponding intensity modulation in the light reflected from the sensing film which is then detected by a photodiode. Linear operation is achieved for small measurand-induced phase shifts by tuning the wavelength of the laser source so that the interferometer phase bias is set to the optimum quadrature point.
Figure 2 Sensor output (50µm water-backed PET film) in response to photoacoustic and photothermal signals generated in an ink-Tris absorber (ma=70cm-1). Inset shows complete thermal signal over expanded timescale. Fluence: 0.27mJ/mm2, pulse duration: 5ns, repetition rate: 16 Hz.
Experimental set-up and procedure: Figure 2 demonstrates the dual sensing ability of the system showing the photoacoustic and photothermal signals generated in an ink/Tris solution of absorption coefficient µa=70cm-1 over different timescales. Figure 2 shows the short duration (400ns) photoacoustic signals. The step decrease, immediately following the initial thermoelastic wave is due to the initial heating of the target by the laser pulse and can be regarded as the onset of the rising edge of the thermal wave. This very slow increase in the thermal signal can be seen more clearly in the inset which shows an expanded timescale graph.
Performance: The acoustic system sensitivity was obtained by comparing the sensor output to a calibrated 25MHz PVDF membrane hydrophone and was found to be 140 mV/MPa with an acoustic noise floor of 2 kPa over a 25 MHz measurement bandwidth and 30 averages. The dc thermal system sensitivity was established by placing the sensor head in a water bath and recording the sensor output as the temperature was varied. A calibrated thermocouple placed immediately adjacent to the sensor head was used as a reference. The dc thermal system sensitivity was found to be 32 mV/ºC with a thermal noise floor of 6.3 x 10-3°C, also over a 25 MHz measurement bandwidth and 30 averages. By varying the temperature over 25°C, it was possible to observe a maximum and minimum of the interferometer transfer function (indicating a phase shift of π rad) giving a temperature (phase) sensitivity of 0.13rad/°C.
Applications: In vivo measurement of tissue optical properties – Analysis of the amplitude and temporal characteristics of the photoacoustic and photothermal signals can yield the optical properties of the target tissue and can be used to discriminate between different tissue types. For example, photoacoustic spectroscopy has been used to characterise arterial tissues based upon the strong preferential absorption in atheroma at visible wavelengths and we are currently investigating the use of photothermal methods for the detection of cancers. The flexibility and small size of the probe offers a means of implementing these techniques in vivo in a minimally invasive form such as via a biopsy needle, endoscope or catheter. Additionally the use of polymer film deposition techniques enables the sensor to be batch fabricated at low unit cost for disposable use to avoid cross infection.
Laser ablation studies: The probe has application in fundamental studies of laser ablation where there is a need to measure the acoustic and thermal transients generated and relate them to the degree of disruption produced in the target. For example, in biomedical laser ablation processes such as laser angioplasty the acoustic transients generated can produce damage to the vessel wall beyond the ablation site and it has been suggested that the resulting increased trauma to the vessel may play a role in stimulating restenosis. It would be useful to quantify such photomechanical effects and additionally any photothermal response by the direct measurement in vivo of the laser-induced acoustic and thermal transients and relate them to the observed physiological response.
Non-destructive testing (NDT): The probe may also act as a miniature all-optical laser ultrasound transmitter and receiver for laser NDT/ND evaluation applications (e.g. flaw detection in engineering structures) that require an inexpensive integrated probe capable of making same-side coaxial measurements. The flexible nature of the optical fibre downlead and its very small diameter (0.25mm) provides a means of making measurements in locations that are difficult to access such as pipes or small cavities. The inexpensive nature of fabrication allows the probe to be considered an inexpensive consumable that can be used in hostile environments where there is a risk of damaging expensive piezoelectric transducers. Since the probe is electrically inert it can be safely deployed in flammable/explosive environments where there is a risk of ignition from a electrical sources and used in electromagnetically noisy environments.
References
- Beard, PC, Perennes, F, Draguioti, E, and Mills TN (1998): An optical fibre photoacoustic-photothermal probe, Optics Letters 23(15), 1235-1237. Download PDF file
- Laufer, JG, Beard, PC, Walker, SP, and Mills, TN (2001): Photothermal determination of optical coefficients of tissue phantoms using an optical fibre probe, Physics in Medicine and Biology 46, 2515-2530. Download PDF file
- Laufer, JG, Beard, PC, and Mills, TN (2002): Comparison of the photothermal sensitivity of an interferometric optical fiber probe with pulsed photothermal radiometry, Review of Scientific Instruments 73(9), 3345-3352. Download PDF file
Laser diode excitation system
An important capability of photoacoustic imaging is the ability to spectroscopically identify chromophores such as oxy and deoxyhaemoglobin by imaging at multiple excitation wavelengths, usually sourced from Q-switched Nd:YAG pumped OPO based systems. However the large size, low pulse repetition frequencies (tens of Hz), expense, power and cooling requirements of these systems limits their practical biomedical application, particularly within a clinical environment. For this reason we are developing a new generation of photoacoustic excitation sources based on the use of laser diodes. These are readily available in a range of visible and NIR wavelengths, inexpensive and compact. The relatively low peak powers available from laser diodes (to avoid facet damage) might at first suggest they would provide insufficient photoacoustic signal amplitudes. However the ability to arbitrarily modulate their temporal output at high frequencies provides an opportunity to overcome this through the use of novel time and frequency domain modulation strategies. For example, the much higher pulse repetition frequencies (PRF) that can be obtained using laser diodes (several kHz compared to the tens of Hz typically available from OPO systems) enables a large number of acquisitions to be rapidly signal averaged to improve SNR. Further improvements may be possible by optimising the pulse duration for specific penetration depths. For example, much of the higher frequency content of a photoacoustic signal generated at depth by the absorption of a 10ns pulse from a fixed pulse duration Q-switched laser may be lost due to frequency dependent acoustic attenuation in tissue. Under such circumstances it would be more efficient to deposit the laser energy over a longer pulse duration (consistent with the usual requirements of stress confinement) to preferentially excite a photoacoustic signal with a lower frequency content that will be attenuated less. Our preliminary work in this area suggests that by employing these strategies and combining a reasonable number (10) of high peak power laser diodes, it will be possible to obtain SNRs comparable to those obtained using Q-Switched Nd:YAG pumped OPO systems.
References
- Allen, TJ, and Beard, PC (2006): Pulsed NIR laser diode excitation system for biomedical photoacoustic imaging, Optics Letters 31(23), 3462 - 3464. Download PDF file
- Allen TJ, Cox BT, and Beard PC, Generating photoacoustic signals using high peak power laser diodes, Proc. SPIE 5697, pp233-242, 2005 download PDF file
- Allen TJ and Beard PC, Development of a pulsed NIR multiwavelength laser diode excitation system for biomedical photoacoustic applications, Proc. SPIE Vol. 6086 60861P-1-9, 2006
|
|